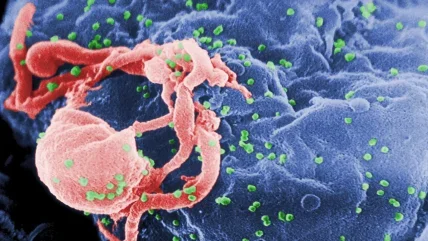
Right now, Ania Fryszkowska and Kevin Maloney, principal scientist and the head of the biocatalysis group at MSD, respectively, are adding a new link to a chain of technological breakthroughs that has shaped human civilisation over millennia. As profound as that sounds, the basis of their work has shaped life itself for far longer. They’re manipulating – even programming – the catalysts behind the metabolic processes that sustain us.
But we should start with some specifics. MSD’s biocatalysis group has developed a new, more sustainable process for synthesising Islatravir, an experimental treatment for HIV. That would be laudable on its own, but something much more impactful is happening behind the active pharmaceutical ingredient (API). By engineering proteins with specific capabilities and using them to integrate multiple enzymatic reactions into a single biocatalytic cascade, the MSD team has synthesised the drug with less than half the steps and operations required for previous chemical routes, while still achieving a huge improvement in atom economy.
Biocatalysis is not a new approach, but the use of nine enzymes in concert, and without any supplemental chemical catalysts, is almost unprecedented, as are some of the transformations MSD has achieved through those interactions.
The implications for the future of pharmaceutical design and manufacturing are tantalising. “Every step, every operation adds complexity to a process,” says Maloney, a more traditional synthetic-organic chemist by training, “and the more steps you take, the more material you need, the more solvent you need – the more everything. We went from 16 steps right down to – depending on how you want to count this – one to three. That correlates to everything. You’re going to get a higher yield; you’re going to generate significantly less waste; you’re going to have lower costs, quicker turnaround times and less time in the manufacturing plant.”
This remarkable improvement in sustainability and efficiency comes down to a set of properties that are unique to enzymes, amid a flurry of advances made in our understanding of them that blur and befuddle the distinctions we make between nature and technology.
One-pot suits all
“Enzymes evolved to work together in tandem,” explains Fryszkowska, the second author on the Islatravir paper, “so they will only perform the reactions that they were designed to perform.” At the same time, they all function in the same mild reaction conditions, which means they’re uniquely suited to be used in ‘one-pot’ cascades that don’t require high temperatures or extra operations like isolations or solvent changes.
Whereas previous routes for the chemical synthesis of Islatravir required costly and labour-intensive interruptions, such as purifying intermediate compounds and modifying molecules to include protecting groups that stop them from reacting to the wrong catalysts, the product of each step in a true biocatalytic cascade simply becomes the substrate for the next transformation – effectively automating the entire process. As such, there’s only one isolation in the Islatravir cascade: Islatravir itself.
But enzymes didn’t evolve for industrial use. To do something so specialised on an appropriate scale, they require a bit of guidance. As explained in the boxout on page 30, biocatalysis has played a part in many of the most impactful scientific and technological advances in human history, but perhaps nothing has been as astounding as Frances Arnold’s Nobel Prize-winning work on directed evolution.
Given some of its philosophical implications, Fryszkowska does well to summarise what directed evolution means in practice. “In a similar way to how we programme our software to store our information,” she says, “biology stores a function in a gene sequence. Ultimately, that’s translated to a protein sequence, and then that protein will have that function. This is what Frances Arnold’s discovery allows us to understand – that we can modify the gene sequence and therefore modify the function.”
Working backwards from Islatravir, the team identified enzymes able to break it down into simpler molecules. This was possible because, like numerous other antiviral and anticancer drugs, Islatravir is a nucleoside analogue, and, according to the reversible bacterial nucleoside salvage pathway, can be constructed from the intermediates left behind when it is broken down.
“Because a lot of these reactions are in equilibrium,” explains Maloney, “you can actually explore the synthesis in the reverse direction. So, you take the API, you throw in a phosphorylase and you see if it breaks the carbon-nitrogen bond. Once you have a principle of that, you then realise you can probably force it to go in the other direction.”
For the most part, however, wild-type enzymes are poorly suited to either synthesising or degrading inorganic molecules. That’s what makes directed evolution so important. In this case, the team took enzymes that showed any activity towards Islatravir and its intermediates along the nucleoside salvage pathway, and made countless tiny alterations to try and optimise them for catalysing a synthesis.
“We made thousands of [different] DNA sequences and then produced, as a result, thousands of protein variants that differed in their sequences a little bit, but were very similar to the original proteins,” Fryszkowska continues. “Then, we screened which of those proteins performed the best in our reaction and iterated. That’s probably the key difference between biocatalysis and a chemical approach. As we develop the process, we also, in parallel, engineer those proteins and improve them. So, we engineer the biology and improve the chemistry at the same time.”
9
Enzymes that were used in the Islatravir cascade.
5
Enzymes used in the Islatravir cascade that were engineered to synthesise the API.
MSD
“Every step, every operation adds complexity to a process, and the more steps you take, the more material you need, the more solvent you need – the more everything.”
Kevin Maloney
‘Improve’ might actually be an understatement. Eye-popping multiplications bubble up all over the Islatravir cascade paper. A variant of purine nucleoside phosphorylase was engineered with a staggering 350-fold improvement in activity. “And with kinase, the activity we initially discovered was below 1% conversion,” adds Fryszkowska, “but we were able to evolve the enzyme to give 100%-conversion under very low enzyme loading. So those improvements were even greater than 100-fold.”
Fryszkowska makes her distinction between bio and chemocatalytic approaches to synthesising APIs advisedly. The success of the Islatravir project has effected a genuine cultural shift in how MSD approaches drug discovery and manufacturing.
51%
Yield of Islatravir from the entire biocatalytic cascade.
36%
Comparatively, the yield of Islatravir from the best available chemocatalytic route, which is considerably longer and more labour-intensive.
MSD
As his position as the head of biocatalysis might indicate, Maloney realised the potential before many of his colleagues elsewhere in the company, but his experience helps demonstrate how a similar change in thinking may soon sweep the industry as a whole.
Culture change
“We didn’t start out saying, ‘Let’s come up with a single cascade from commodity chemicals to MK-8591’,” he admits, referring to Islatravir by its company code. “We looked at it more as, ‘Let’s fix this problematic reaction’, and then, as we really saw the power of enzymes, we were like, ‘Wait, we can do all of this with enzymes – this could change the way we look at all our goals’.”
That comes back to the aforementioned nucleoside salvage pathway, which is used by bacteria to recycle genetic material. In order to utilise it for Islatravir, the team needed to break and make one particularly stubborn carbonnitrogen bond between ribose and 2-fluoroadenine. Maloney referred to it earlier, but his phrasing glossed over just why it was so important.
“I don’t want to say that bond can’t be made another way,” he clarifies, “but we never found a good way to make it with complete selectivity through synthetic-organic chemistry. But nature makes a ton of these nucleosides, and they make only one diastereomer, so they make that bond with complete control. And as we found this phosphorylase, as we understood this CN bond formation, I think that’s when the team started to realistically dream and say, ‘Why can’t we do this? Nature can do it; nature does it – we all have nucleosides in our DNA.’”
That’s not quite how Maloney was trained to do chemistry. Were he trying to synthesise Islatravir when he was at graduate school, he would have gone straight to the lab to try and chemically formulate the type of ribose phosphate that broke the carbon-nitrogen bond between the ribose and 2-fluoroadenine. He contrasts that directly with Fryszkowska’s approach. “She would come in and say, ‘Let me take the API and let me look at the reverse direction’,” he recounts. “‘And if we find an enzyme that does it at 1%, then we can go to evolution and we can find a way to force the equilibrium in the direction we want.’”
That’s just what they did; from there, the glycosylation cascade began to take shape. “Once we had all the enzymes,” says Fryszkowska, “we started putting stages together in the reaction, realising that it was getting better and better, and becoming more and more efficient.” The two reversible enzyme-catalysed reactions in the glycosylation cascade need to happen concurrently in the presence of the third enzyme, sucrose phosphorylase, to push past equilibrium limits and achieve a higher yield. The addition of a fourth enzymatic aldol reaction, with a compatible temperature and pH requirement, introduces further synergies, and lowers the material requirements. (There are also two enzymatic operations that take place before the glycosylation cascade.)
“We found we could build complexity in a single part in an almost effortless manner,” Fryszkowska continues. “The glycosylation cascade works beautifully with four enzymes acting in the same process at the same time.”
And it’s the cascade of benefits that flow from biocatalysis – from the efficiency improvements and cost reductions that come from needing fewer materials and operations, to the sustainability and safety benefits of mild reaction conditions and fully renewable catalysts – that have turned heads at MSD. “Now, when we think about new molecules,” says Fryszkowska, “we brainstorm on both chemo and biocatalytic approaches hand-inhand. That, for me, is quite a remarkable change – for the entire department, all the chemists, to embrace this as a viable option and feel able to propose and make connections based on two different models of catalysis.
“We need to be thinking about the most efficient ways of accessing all these new molecules and modalities, both in processing and discovery, which means tapping into whatever type of technology will help us to make them faster and better.” And for all the possibilities of the internet of things and edge computing, biology still has us licked on automation.
New wave
Ania Fryszkowska describes the pattern of biocatalytic developments that made this discovery possible as a series of waves. The first – fermentation – was a great wash of alcohol, a tipsy continuation of the flood that soused so many mythologies. Few discoveries have had a bigger impact on our minds and lives. It preceded and, some have argued, gave rise to recorded history. Impressive, but, bread notwithstanding, it took approximately 10,000 years for humanity to start putting biocatalysis itself to work for anything much less indulgent than beer, wine or cheese.
Finally, in the early 19th century, Anselme Payen discovered the first of the enzymes that made those reactions possible, and within 100 years, the penicillium fungus had been used to create the very first antibiotic. From there, the frequency of developments in biocatalysis have increased so much that the progress is almost audible.
In Fryszkowska’s second wave, scientists worked out how to isolate and use specific enzymes rather than whole tissues to catalyse reactions – giving them much greater control over the process itself. For pharmaceuticals, penicillin is again the best example: today, penicillin G acylase is used on a multi-tonne scale to produce a range of semi-synthetic antibiotics.
The next set of advances came in the 1980s and ’90s, as Frances Arnold pioneered the use of directed evolution to create enzymes with novel or improved functions – work that has given humanity the power to manipulate and program nature on the most fundamental level.
Progress is now happening so quickly that Fryszkowska can’t keep track of the waveform. “I think now we are in the fourth wave of biocatalysis, or maybe even the fifth,” she says. “But both waves, I would say, are when we no longer replace a singular chemical step, but we replace a large portion or the entire synthesis using biocatalytic cascades.
“Biocatalysis is no longer just a better solution or a greener solution to a challenging chemical reaction, it’s a way to fundamentally rethink how we build the complexity of unnatural compounds. This is the core of our work.”