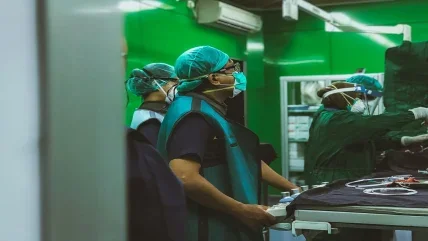
On 2 December 1959, Nobel prize-winning physicist Richard Feynman gave an infamous speech at a meeting of the American Physical Society at Caltech. A lecture on the subtleties of gravity, or the ins and outs of thermodynamics, this was not. Instead, Feynman regaled his audience with the potential of miniature robots in medicine, speaking of tiny machines that would navigate their way through bodily tissues and cavities, performing diagnostics and intricate surgical procedures. The doctor of the future, the physicist argued, would be microscopic and fully capable of being swallowed.
Feynman’s speech is widely regarded as the first public argument for nanotechnology and, given today’s advancements in manufacturing and biological engineering, his words have a prophetic ring to them. Nanoscale engineering is now experimenting with a range of possibilities from tiny spider-like robots that neutralise bacteria in the bloodstream, to microbots that target tumours with precise laser ablations.
The potential for nanotechnology in transforming targeted drug delivery is particularly exciting – especially in cancer treatments, where biologically inspired synthetic materials have birthed exciting new forms of therapies. These can enable drugs to reach tumours faster and with greater accuracy, while allowing oncologists to monitor cellular changes in the patient in real time.
By using these new-found capabilities, drug makers can not only kill cancers more effectively, but also improve upon current forms of chemotherapy that fail to discriminate between healthy tissue and cancerous tumours, causing a range of nasty side effects.
These new therapies are being brought about by a more sophisticated understanding of nano-biological interactions; namely, how tiny devices such as nanotubes can be transported into cells, avoid damaging healthy tissues and be manipulated to carry out specific tasks.
For Chunlong Cheng, senior scientist at the Department of Energy’s Pacific Northwest National Laboratory, a particularly exciting area of this research is in bio-inspired functional materials, the art of imitating and recreating complex sequences of molecules and tissues found in biological systems.
More specifically, Cheng is dedicated to studying proteins, developing synthetic polymers that mimic how proteins function in the body, enabling them to be used in a variety of applications such as water separation, molecular sensing and, most importantly, as transportation devices for drugs at a cellular level.
“Nature can make a lot of amazing things,” Cheng says, referring specifically to porous cell membranes, and their capacity to absorb and hold chemical compounds. Unfortunately, as Cheng explains, nature is also hard to break down, and convincing it to accept synthetic tissues deep inside the body’s defensive cellular wall structures is a complex and intricate task.
“The goal is to make biologically inspired materials that are similar to natural materials, but more stable, so they can then be used inside the body for different applications,” Cheng explains.
From small beginnings
The first nanomolecules used for drug delivery were liposomes – capsules made up of layers of lipids that function in a similar manner to membranes. These structures housed the first ‘nanodrug’, doxorubicin (Doxil), which was approved in 1995 and developed specifically to kill cancers – specifically metastatic ovarian cancer and Aids-related Kaposi’s sarcoma.
In recent years, however, the focus of research has shifted towards exploring the potential of peptoids to deploy therapies at the cellular level. These Artificially created sequences designed to mimic proteins in the body, such as peptides and amino acids, have unique characteristics that make them highly advantageous as carriers for therapies, contorting into structures that mimic patterns in cell biology and making them ideal containers for DNA assay and drugs.
Some of these devices benefit from the tendency for blood vessels wrapped around tumours to leak, a phenomenon known as the enhanced permeability and retention (EPR) effect. This allows nanoparticles to infiltrate the tissue and cling on due to relatively poor drainage. Other types, meanwhile, have tiny molecules or antibodies on their exterior that bind onto cancer cells, allowing them to pass through the wall of the cell membrane.
These advantages are explored in a paper released in 2019 in Frontiers in Pharmacology, in which the authors discuss recent advances being made in the sphere of nano-based therapies for cancer treatment. In particular, they argue that current research and development on protein-based nanoparticles for cancer theranostics holds great promise.
As the authors note, one of the early drugs showing promise in the field is Abraxane, recently approved by the FDA, which combines the traditional chemotherapy drug paclitaxel with a protein called albumin. While still in early stages of development, the combination has been shown to afford higher dosing than regular paclitaxel with little or no side effects in some trials.
For Cheng, the rise of these new and exciting forms of treatment invariably come with a headache; namely, how to design these complex sequences of proteins at a miniscule level and ensure that they can carry drugs through the body.
“The question was: ‘How can we make this material into a nanomaterial?’, because then we can not only control the composition, [but] we can also control its size and shape,” Cheng explains. “By using the peptoids, we can programme this nanomaterial for a very specific application.”
Ideas begin to bloom
Recently, Cheng and his colleagues have been designing nanoflower-like particles from peptoid sequences. By building up sheets of peptoids, they have successfully created tiny flower-like particles measuring up to 150nm in size, roughly a thousand times smaller than the width of a piece of paper.
After adding probes to these structures, the researchers were able to trace their progress through cells, while adding fluorine to allow them to escape from cellular traps that frequently impede the drug delivery process.
Importantly, the experiment was devised to see if these structures could evade some of the pitfalls that have plagued numerous other experiments at the bioinspired nanoscale level. One of these is cytotoxicity: a toxic reaction in cells that is caused when nanoparticles puncture through membranes, disrupting and damaging these structures, eliciting a defensive response from tiny proteins that regulate cells, called cytokines. If these structures are unnaturally disturbed or imbalanced, this can damage or destroy cells, triggering dangerous side effects in patients.
As Cheng explains, the key challenge with this experiment and all synthetic construction schemes is to engineer the chemical equivalent of a Trojan horse, tricking the cell’s defence mechanisms into perceiving these artificial invaders as natural allies.
In this particular lab test, not only were the nanoparticles able to escape from endosomes – membrane compartments that can trap and prevent nanocarriers from delivering drugs – but they exhibited minimal toxicity, making them attractive as cellular drug carriers.
As such, the nanoparticles successfully entered the cell, permeating the membrane and swimming through its interior chamber, before being captured and released by endosomes. Theoretically, were this operation to be carried out within a cancerous cell, the particles could shed their protective peptoid coating and administer drugs to kill a tumour.
Walk before you run
Going forward, Cheng is quick to point out that the implementation of this technology in clinical trials is some way off. “We are still in the very early stages with this project and there are lots more tests that need to be conducted,” he stresses.
Key challenges remain when it comes to manoeuvring these devices through the body, particularly as these nanomaterials can change and mutate as they enter the bloodstream, potentially causing a dangerous reaction in the patient.
“Most peptides are made by the body, so they are biocompatible,” Chengs explains. “But that means that they can degrade and trigger a sequence. The problem is that we don’t know if those sequences will be highly toxic. They are really hard to predict.”
Despite these downsides, biocompatibility is by its very nature a double-edged sword, bringing with it a range of exciting opportunities that can be unlocked through further research. Not only do these tiny protein-based mechanisms possess the potential to target cancer with greater accuracy, freeing healthy cells from harm – and killing tumours that would otherwise be inaccessible – but they also have the ability to respond to a variety of stimuli, such as temperature or acidity.
Apoferritin – a protein found in the intestinal mucosa membrane – is composed of self-assembling peptides that form a cage-like structure that can be unravelled according to PH levels, making it an exciting, if unfulfilled, case study as a nanocarrier for drug delivery.
“The key advantage of these materials is that they are highly tuneable,” Cheng says. “So, in the future, we can direct these peptoids inside [the body] to a specific location and they can be programmed in sequences to do crucial tasks, like deliver certain drugs or perform diagnostics. Because of this they have huge potential.”
While there is work to be done, particularly on how these biologically inspired creations respond within the body and how they interact with tumours on a cellular level, nanomaterials have the potential to revolutionise how treatments and diagnostics are performed. These miniscule, intricately developed materials could yield the biggest developments in the fight against cancer.
150nm
The size of the tiny flower-like particles Cheng and his colleagues are able to create by building up sheets of peptoids.
Department of Energy